That’s the caldera of Santorini Volcano behind this beautiful little poser.
Now put on your hard hats — it’s time for a little chemistry.
Very little.
In fact, just this: “What do you get when you remove the oxygen (chemical symbol O) from H2O?”
Okay, so there’s really no need for special gear (unless you want to follow a working geologist out into the field to collect and study some of the world’s oldest rocks).
You get H2 — hydrogen — of course.
Believe it or not, this answer is closely tied to the origin of life on Earth. So is volcanism: source of the heat energy powering that and other chemical reactions necessary for emerging life.
Rocks — solid as well as molten — are involved, too, in complex ways that range from providing nutrients to acting as templates and catalysts. (Hazen; Guttenberg et al., 2017, 2021; NASA, 2020b; Walker)

Ganymede looks very familiar, but it’s really not much like our Moon otherwise. (Image: Twitter)
In fact, rocks are so important that NASA is focusing its search for local ET to icy moons Europa (Jupiter) and Enceladus (Saturn), instead of on the photogenic Jovian orbiting snowballs Ganymede and Callisto.
Why?
Because subsurface oceans on Europa and Callisto probably are in contact with surface rock, not in between ice layers, as they appear to be on Ganymede and Callisto. Chances of finding life are a little better. (NASA, n.d.; 2020b, 2021c, 2021d)
Spacecraft passing close to Europa have even detected evidence for hydrothermal vents, like these on Earth (though no sign as yet of accompanying life):
You’ll need more than a hard hat down here!
The big puzzler is whether there’s a direct connection between geology and the evolution of life, and if so, what it is exactly.
That must go unanswered, but in this chapter we can get a general idea of evolutionary events on young, fiery Earth that must have occurred before LUCA (the Last Universal Common Ancestor of modern life) appeared.
Volcanism and continental shields
To bring things up to date:
The Earth [after the Moon-forming impact] began at rock-vapor temperatures and gradually cooled to habitability over 20–120 million years. By the time it was habitable, its mantle was mostly solid, but a few 100 K hotter than present. Volcanic rocks erupted to the surface and hydrothermal water circulated through hot rocks. At least some continental mass was exposed above sea level.
— Sleep (see reference list at end of post)
As we saw last time, the geology of Earth right after its magma ocean crusted over and cooled a bit was very different from today.
Using hard-hat-style geochemistry and geophysics, experts have figured out some of the details of how our planet took on its modern mode (plate tectonics).
Since we’re looking at evolution that ultimately led to cats, we don’t really need to know the minutiae of it all (some of which are controversial, anyway).
You can take my word for it that there was lots of volcanism going on while precellular life emerged, as well as the presence of lots of rocks above and below sea level.
Or, if you’re curious, here are two talking heads, describing some highlights in easily understandable terms, first in the classroom and then out in the wilds of Wyoming.
Enjoy! Or feel free to skip over this to the next section, where we get back to life’s early history again.
Note: At the end of this post, there is also a bonus video of what happens when a biologist and National Geographic get together to visit a continental shield in Guiana that is VERY different from the one discussed above, which is in Greenland and parts of Canada and the US.
Hydrothermal “hatcheries”
Earlier, we watched life thriving as seawater circulated through modern hydrothermal vents in a Caribbean deep sea trench.
Similar processes must have occurred in the oceans of Earth shortly after the planet’s formation 4.5 billion years ago. That’s just how geochemistry and geophysics work.
Evidence from ancient zircons strongly suggests that the planet did have oceans and running water that early. Perhaps, as the cooling mantle outgassed its nebular water, the resulting steam condensed into rain drops after Earth’s temperature went down a bit, and basins all over the world filled up. (Zahnle et al.)
The hydrological cycle had begun, but those alien-looking critters in the hydrothermal vent video weren’t around yet.
These shrimp and their companions may look primitive, but they’re actually highly evolved — yes, even little cellular things that are part of this ecosystem but don’t show up on camera.
To get technical for a moment, those tiny shrimp, etc., represent what many biologists call Eukarya (a domain that also includes every form of life you can see without magnification). The little cellular things belong to the other two Domains of Life — Archaea and Bacteria.
None of these thermophiles — “heat lovers” — physically resembles the first precellular life forms, which I imagine (probably incorrectly) as a sort of slimy goo on and/or in the rocks.
Why?
Because they aren’t direct descendants of the slimy goo.

Of course, something metaphysical can neither be proven nor ruled out. (Image: mayavase via Wikimedia)
Metaphysics aside, there seems to be overall scientific consensus that what I call slimy goo came first; after that, at some point, came LUCA, the Last Universal Common Ancestor, which already had well-developed cellular “stuff” (Walker et al.); and then from LUCA came the Three Domains, whose early forms left behind those famously ancient fossils. (Schopf; Walker; Walker et al.; Zahnle et al.)
The tricky part is identifying the huge evolutionary steps needed to transform slimy goo into LUCA in less than 500 million years (that is, the relatively short span of geological time, per Zahnle et al., between Earth’s cooling down to habitable temperatures and the moment when cyanobacteria, very similar to ones today, died 3.5 billion years ago, leaving their remains in an excellent fossilizing environment on what is now the western Australian craton).
It’s quite a problem for the boffins, especially because the fossil record suggests that major steps in evolution — like developing body structural components — often happen very slowly. (But this is very controversial, as we’ll discover later in the series.)
However, I do get an impression of general scientific agreement on one point, anyway: whatever its history, precellular life definitely wasn’t complex.
It probably lacked even a protective membrane at first! (Mulkidjanian et al.)
Hydrogen could satisfy its needs, and there was plenty of that available wherever water circulated through rock near magma. (Sleep; Sleep et al.)
Hydrothermal vents must have been almost everywhere, given the abundance of water, rocks, and ongoing volcanism at this point in the planet’s history.
As I understand it, planet-wide hydrothermal circulation disturbs the delicate, sterile balance of Earth’s rock, water, and carbon cycles. In short, it causes a chemical gradient of hydrogen that the first living organisms could have used as energy. (Sleep; Sleep et al.)

Still at Santorini, but the cat might be a different individual. (Image: Kathryn Burrington, CC BY-NC 2.0)
Of course, early Life didn’t put on a hard hat and reason out things like “CO2 + 4H2 –> CH4 + 2H2O,” producing methane (which soon turned Earth’s runaway greenhouse atmosphere into a yellow haze, per NASA 2020b), or “2CO2 + 4H2 –> 2CH2O + 2H2O,” producing acetate, which could provide the hungry little things with organic molecules as well as energy. (Both of these equations are from Sleep and Sleep et al.)
No, Life simply found a good “diner” — early hydrothermal springs on the seafloor, perhaps, or a geothermal area on land — and started chowing down on the daily special: H2 and other goodies, like boron (warning: this links leads to a hard-hat zone, but hey, it describes possible chemical ingredients for ancient life on Mars!). (Mulkidjanian et al.; Sleep; Sleep et al.)
Wait. On land?
Everyone knows life originated in the sea! Look at the hydrothermal vents!
True, but there are important problems with the notion that life on Earth only evolved beneath the waves.
- For one thing, some sort of Late Heavy Bombardment was still happening at the time, with huge space rocks coming in; the biggest impacts could boil oceans. After that, incredibly hot rain would then fall, and surface life would only become possible again after a few thousand years. The likeliest long-term survivors of such catastrophes would be microbes able to withstand high temperatures — thermophiles — and also wearing “hard hats,” i.e., living in Earth’s crust just as some unrelated microbes do today. (Sleep)
- For another, those hydrothermal vents are very deep. Their residents live off chemical energy, which works but isn’t very productive. (Sleep; Sleep et al.) If all life began down there, how could some of those primordial microbes work out a way to convert sunlight into energy — photosynthesis — so they could take over the world? (Corsetti et al.; Schopf)
The first microbe that could dispense altogether with H2 proliferated with its productivity increasing by a factor of thousands above the previous total primary productivity of the Earth. It occupied all suitable environments on the scale of years to thousand of years. Its descendents held most of the tickets in the subsequent evolutionary lottery.
— Sleep
- Too, here’s something you might not know: The popular idea that the chemistry of our cells is like that of seawater is a misconception.
Mulkidjanian et al. get into this, but you’d want a hard hat to read the whole paper, although it’s pretty interesting.A plain-language interpretation of the relevant nontechnical part by this layperson is that, although there are similarities, there are also important differences between cell biochemistry in all living things (including us) and seawater.
This undercuts the idea that life’s very first appearance was intimately connected with the ocean.
The researchers also describe some really weird chemistry in parts of all modern cells.
This is identical to a geochemical environment that ancient life would have encountered in certain parts of land-based volcanic hydrothermal systems, like those known today at Yellowstone National Park and at two geothermal power-generating sites: The Geysers, in California, and Larderello, in Italy.
Warned you about that hard hat! But remember — the first precellular life was a thermophile survivalist, and this sort of thing was its battlefield. According to these researchers, that “vapor dominated zone” is where the slimy goo could evolve. “Meteoric water” is just rain and ground water; “exhalations” are surface fumaroles; and “thermal waters” are hot springs. (Image: Figure 1, Mulkidjanian et al., PNAS Open Access)
In fact, Mulkidjanian’s team note that the world’s largest known vapor-dominated zones are underneath The Geysers and Larderello.
Relax. You can visit all of these places today without fear of a new living horror of some type oozing out at you from a crack in the ground (dibs on the movie idea, though!).
For seriously hard-hat-chemistry reasons, the researchers report that, under current conditions on Earth, this vapor zone is much too acidic to support Life; nothing is evolving down there now.
It was only possible back in the day when Earth’s atmosphere was a runaway greenhouse, loaded with carbon dioxide.
I have no idea how many scientists accept this hypothesis, since it can’t be tested in the field now and, per Sleep, such early forms of life would leave little evidence in the geologic record.
Still, the paper has hundreds of citations and is published in a reputable journal. Take this interesting idea for what it’s worth.
Because the geothermal-origin option requires a smoggy Earth atmosphere, life must have evolved before plate tectonics began, if it’s correct.
Plate tectonics locked up all that excess atmospheric carbon dioxide in our planet’s mantle, leaving the balanced carbon cycle we know today. (Sleep)
The other alternative — life originating at hydrothermal vents — is much less time limited, despite its problematical parts. And Time usually is on Life’s side.
Perhaps both ideas are right.
Having more than one type of deeply buried “hatchery” certainly would improve the chances for precellular life surviving Earth’s very hostile environment long enough to get established.
We probably will never know for sure what happened.
As Dr. Sleep points out in his paper:
Nascent life competes with nonlife (Nowak and Ohtsuki 2008). There is selection both for efficient gathering of resources and for faithful reproduction. Once the fidelity of reproduction crosses a threshold, life wins. The population explosion colonizes all connected environments on a time scale of years to thousands of years. It is highly unlikely that
the Earth’s meager geological record preserves this event.
So. Once life got started, what happened next?
Somehow, slimy goo evolved into LUCA, and the Three Domains of Life eventually appeared. Members of one of those domains won the evolution lottery by inventing photosynthesis and then poisoned the world with their excessive waste: oxygen.
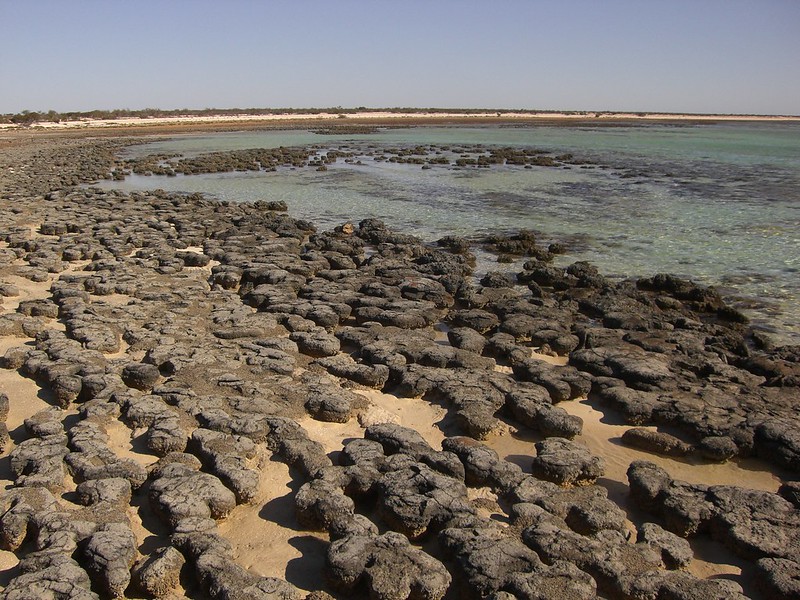
“We did it, and we’re proud!” — Stromatolites. (Image: Pat Scullion, CC BY-ND-NC 2.0)
This, and more, is in the next chapter.
Coda:
Before we get into that, let’s see how a biologist views some of the oldest rocks on Earth.
As it turns out, he doesn’t even notice them.
He’s more interested in frogs. (There are also five wild cats in this ecoregion, including jaguars and pumas.)
But those views of the famous Venezuelan table lands are breathtaking!
Feel free to get your own “Lost World on, too.
Sir Arthur Conan Doyle was wrong, though, about the tepuis being volcanic and only going back to dinosaur days.
They are actually Precambrian in age, about 1.7 billion years old, and part of the Guiana Shield. This craton is one óf about thirty-five ancient fragments of continental crust, and one of the youngest, believe it or not.
Craton sections in parts of Canada and Greenland go back some 4 billion years or more and are considered the oldest by many, though not all of the writers whose papers I’ve read. (Carlson et al.; Gradstein et al.; Palin and Santosh)
Featured image: mikjáll, CC BY-ND 2.0.
Sources:
Carlson, R. W.; Garçon, M.; O’neil, J.; Reimink, J.; and Rizo, H. 2019. The nature of Earth’s first crust. Chemical Geology, 530: 119321.
Corsetti, F. A.; Olcott, A. N.; and Bakermans, C. 2006. The biotic response to Neoproterozoic snowball Earth. Palaeogeography, Palaeoclimatology, Palaeoecology, 232(2-4): 114-130.
Doolittle, W. F., and Brown, J. R. 1994. Tempo, mode, the progenote, and the universal root. Proceedings of the National Academy of Sciences, 91(15), 6721-6728.
Falkowski, P.; Scholes, R. J.; Boyle, E.; Canadell, J.; and others. 2000. The global carbon cycle: a test of our knowledge of Earth as a system. Science. 290: 291–296.
Fitch, W. M., and Ayala, F. J. 1995. Preface. Tempo and Mode in Evolution: Genetics and Paleontology 50 Years After Simpson. Washington: National Academy Press.
Gradstein, F. M.; Ogg, J. G.; and Hilgen, F. G. 2012. On the geologic time scale. Newsletters on Stratigraphy. 45(2):171-188.
Guttenberg, N.; Virgo, N.; Chandru, K.; Scharf, C.; and Mamajanov, I. 2017. Bulk measurements of messy chemistries are needed for a theory of the origins of life. Philosophical Transactions of the Royal Society A: Mathematical, Physical and Engineering Sciences, 375(2109), 20160347.
https://royalsocietypublishing.org/doi/full/10.1098/rsta.2016.0347
Guttenberg, N.; Chen, H.; Mochizuki, T.; and Cleaves, H. J. 2021. Classification of the Biogenicity of Complex Organic Mixtures for the Detection of Extraterrestrial Life. Life, 11(3): 234.
Hazen, R. M. 2017. Chance, necessity and the origins of life: a physical sciences perspective. Philosophical Transactions of the Royal Society A: Mathematical, Physical and Engineering Sciences, 375(2109): 20160353. https://royalsocietypublishing.org/doi/full/10.1098/rsta.2016.0353
Maizels, N., and Weiner, A. M. 1994. Phylogeny from function: evidence from the molecular fossil record that tRNA originated in replication, not translation. Proceedings of the National Academy of Sciences, 91(15), 6729-6734.
Morton, M. C. 2017. When and how did plate tectonics begin on Earth? https://www.earthmagazine.org/article/when-and-how-did-plate-tectonics-begin-earth/
Mulkidjanian, A. Y.; Bychkov, A. Y.; Dibrova, D. V.; Galperin, M. Y.; and Koonin, E. V. 2012. Origin of first cells at terrestrial, anoxic geothermal fields. Proceedings of the National Academy of Sciences, 109(14): E821-E830. https://www.pnas.org/content/109/14/E821.long
NASA. 2020a. Can we find life? https://exoplanets.nasa.gov/search-for-life/can-we-find-life/ Last accessed July 12, 2021.
___. 2020b. Life in our Solar System? Meet the neighbors. https://exoplanets.nasa.gov/news/1665/life-in-our-solar-system-meet-the-neighbors/ Last accessed July 12, 2021.
___. 2021. NASA selects 2 missions to study “lost habitable” world of Venus. https://www.nasa.gov/press-release/nasa-selects-2-missions-to-study-lost-habitable-world-of-venus Last accessed July 12, 2021.
___. 2021a. Then there were 3: NASA to collaborate on ESA’s new Venus mission. https://www.nasa.gov/feature/then-there-were-3-nasa-to-collaborate-on-esa-s-new-venus-mission Last accessed July 12, 2021.
___. 2021b. Venus overview. https://solarsystem.nasa.gov/planets/venus/overview/ Last accessed July 12, 2021.
___. 2021c. The searchers: How will NASA look for signs of life beyond Earth? https://exoplanets.nasa.gov/news/1681/the-searchers-how-will-nasa-look-for-signs-of-life-beyond-earth/ Last accessed July 12, 2021.
__. 2021d. Life in the universe: What are the odds? https://exoplanets.nasa.gov/news/1675/life-in-the-universe-what-are-the-odds/ Last accessed July 12, 2021.
___. 2021f. What’s out there? The exoplanet sky so far? https://exoplanets.nasa.gov/news/1673/whats-out-there-the-exoplanet-sky-so-far/ Last accessed July 12, 2021.
___. 2021e. Mars 2020 Perseverance rover. https://mars.nasa.gov/mars-exploration/missions/mars2020/ Last accessed July 12, 2021.
___. n.d. Europa Clipper: Ingredients for life. https://europa.nasa.gov/why-europa/ingr.edients-for-life/ Last accessed July 12, 2021
Palin, R. M., and Santosh, M. 2020. Plate tectonics: What, where, why, and when?. Gondwana Research.
Prothero, D. R. 2006. After the Dinosaurs: The Age of Mammals. Bloomington and Indianapolis: Indiana University Press. Retrieved from https://play.google.com/store/books/details?id=Qh82IW-HHWAC
Schopf, J. W. 1994. Disparate rates, differing fates: tempo and mode of evolution changed from the Precambrian to the Phanerozoic. Proceedings of the National Academy of Sciences, 91(15), 6735-6742.
Simpson, G. G. 1944. Tempo and Mode in Evolution. New York: Columbia University Press.
Sleep, N. H. 2010. The Hadean-Archaean environment. Cold Spring Harbor Perspectives in Biology, 2(6): a002527. http://m.cshperspectives.cshlp.org/content/2/6/a002527.long
Sleep, N. H., Bird, D. K., & Pope, E. C. (2011). Serpentinite and the dawn of life. Philosophical Transactions of the Royal Society B: Biological Sciences, 366(1580), 2857-2869. https://royalsocietypublishing.org/doi/full/10.1098/rstb.2011.0129
Taylor, S. R., and McLennan, S. M. 1995. The geochemical evolution of the continental crust. Reviews of Geophysics, 33(2): 241-265.
Walker, S. I. 2017. Origins of life: a problem for physics, a key issues review. Reports on Progress in Physics, 80(9): 092601.
Walker, S. I.; Packard, N.; and Cody, G. D. 2017. Re-conceptualizing the origins of life. Philosophical Transactions of the Royal Society A: Mathematical, Physical and Engineering Sciences,375: 20160337.
https://royalsocietypublishing.org/doi/full/10.1098/rsta.2016.0337
Wikipedia. 2021. History of the Earth. ___ Last accessed July 23, 2021.
Zahnle, K.; Schaefer, L.; and Fegley, B. 2010. Earth’s earliest atmospheres. Cold Spring Harbor Perspectives in Biology, 2(10): a004895. http://m.cshperspectives.cshlp.org/content/2/10/a004895.long
2 thoughts on “Volcanism: A Main Character?”